Development of a telescope for cosmic microwave background polarimetry to explore the beginning of the universe
Jan. 10, 2024 | Aerospace Project Research Associate, ISAS people
Research Summary
How did the universe begin? Various astronomical observations have revealed that the universe was once like a hot and dense fireball in its early stage of the history, which is known as the Big Bang. However, the question of “what determined the initial condition of the universe” is still one of the biggest mysteries of astronomy and astrophysics up until now. It is believed that an exponential expansion of the spacetime, the cosmic inflation, has expanded quantum fluctuations into cosmological scales and generated the Big Bang, but its direct observational evidence is yet found. One of the most promising methods to investigate the inflationary era of the universe is the polarization observation of the cosmic microwave background (CMB) *1, the remnant of the Big Bang; it is expected that primordial gravitational waves generated by the inflation imprinted a unique polarization pattern, called primordial B-mode polarization, on the CMB.
The CMB is a millimeter-wave radiation with an almost isotopic temperature of 2.7 K (-270.45℃) across the sky, but there are small temperature fluctuations at the level of 10-5 (30 μK). The intensity of the primordial B-mode polarization is predicted to be even smaller by orders of magnitude. To detect such small signals, we need to separate the CMB and other radiation more precisely, in addition to making the instruments more sensitive and less noisy. In particular, the polarized radiation from the Galactic plane (Milky Way) is much stronger than the polarization of the CMB. Even when we observe the sky at angles far from the Galactic plane, such strong radiation can partially reach the detectors due to diffraction and stray light and contaminate the weak CMB signals. In fact, for the case of the Planck satellite, such contamination with radiation outside the observing direction was estimated to be one of the major sources of the systematic errors [1].
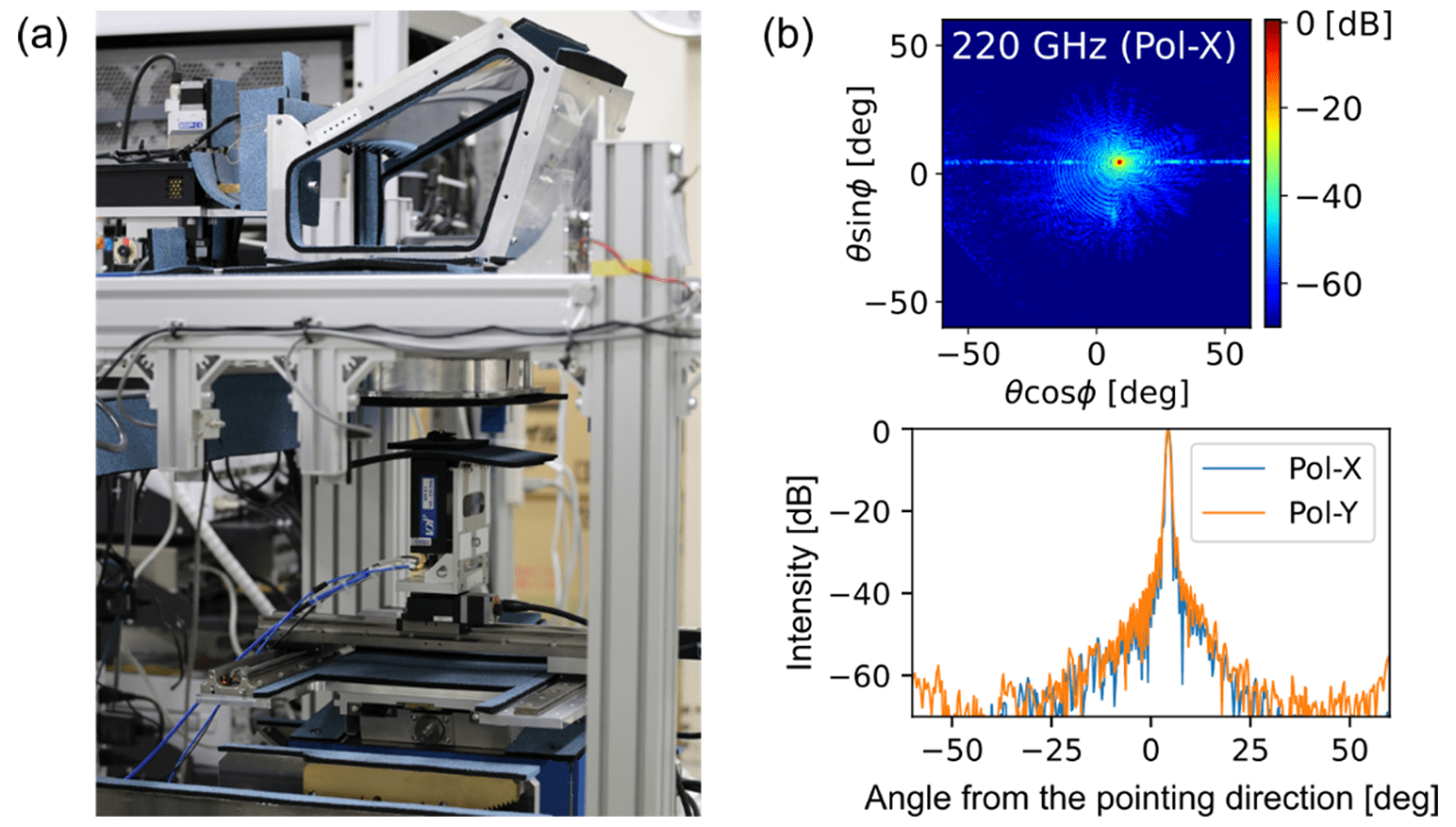
Recent CMB telescopes require a wider field of view to increase the sensitivity and operation at a cryogenic temperature to reduce thermal noise. These requirements make it more difficult to reduce light coming outside the observing direction, which is a major issue in the development of a next-generation CMB telescope. For the JAXA’s CMB observation satellite LiteBIRD, the telescopes’ sensitivity to the radiation at angles far from the observing direction (far sidelobes) is required to be known at the level of -56 dB (0.0003%) [2].
My research focuses on development of new techniques to characterize the optical performance of the CMB telescopes, such as the LiteBIRD Low-Frequency Telescope (LFT) developed at ISAS/JAXA. The LiteBIRD LFT is a reflective telescope with a 400 mm aperture and two reflectors and has a field of view of 18∘×9∘ [2]. In our previous research, we developed a near-field antenna measurement *2 setup and demonstrated that we can characterize the far sidelobes down to the -70 dB (1/10,000,000) level, even at the edges of the field of view (Fig. 1) [3]. Currently, we are establishing a technique to separate far-sidelobe components with different optical paths, so that we can experimentally characterize the stray light inside the telescope (Fig. 2) [4].
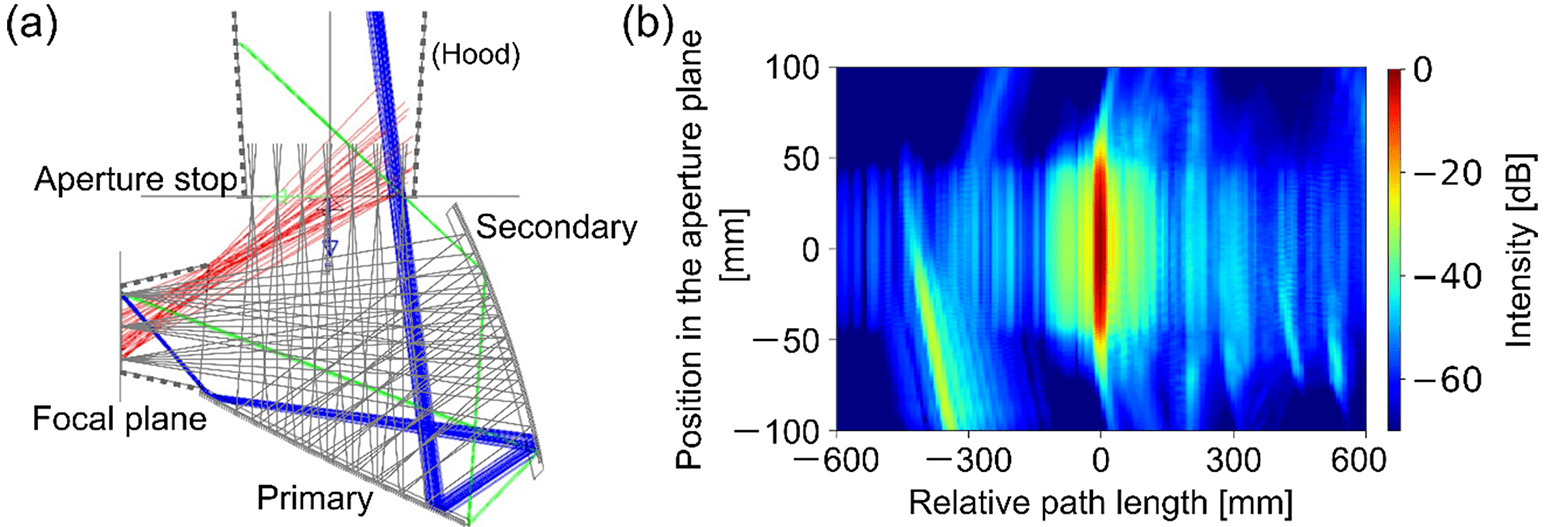
In our experiments so far, we have measured a scaled model of the LFT developed by the JAXA Advanced Machining Technology Group. It precisely scales the geometry of the reflectors designed for the actual LFT to 1/4 size so that measurements at 1/4 wavelength produce the electromagnetic-wave propagation identical to the actual LFT. Based on these experimental results, we are conducting studies on development of the actual LiteBIRD LFT, pursuing further improvements of its optical performance.
- [1] The Planck Collaboration, “Planck 2018 results - I. Overview and the cosmological legacy of Planck,” Astron. Astrophys. 641, A1, 2020, doi: 10.1051/0004-6361/201833880.
- [2] Y. Sekimoto and the LiteBIRD Collaboration, “Concept design of low frequency telescope for CMB B-mode polarization satellite LiteBIRD,” Proc. SPIE 1145310, 2020, doi: 10.1117/12.2561841.
- [3] H. Takakura et al., “Far-Sidelobe Antenna Pattern Measurement of LiteBIRD Low Frequency Telescope in 1/4 Scale,” IEEE Trans. THz. Sci. Tech. 9, 6, 598, 2019, doi: 10.1109/TTHZ.2019.2937497.
- [4] H. Takakura et al., “Straylight identification of a crossed-Dragone telescope by time-gated near-field antenna pattern measurements,” Proc. SPIE 1218052, 2022. doi: 10.1117/12.2627421.
Terminologies
- *1 Cosmic microwave background (CMB) : The light emitted at the time of the Big Bang, around 380,000 years after the birth of the universe, and is the oldest light (electromagnetic waves) that we can observe today. It has been cooled down as the universe has expanded, and nowadays it fills the universe as blackbody radiation in microwave range. It was first discovered by Arno Allan Penzias and Robert Woodrow Wilson in 1964. In the 1990s to 2010s, precise all-sky observation of the CMB was performed by COBE, WMAP and Planck satellites, from which the parameters of the standard cosmological model, such as the energy composition and age of the universe, are precisely determined.
- *2 Near-field measurement method : Optical performance of a telescope can be measured by observing a known calibration source. Because the light from a point source spreads spherically, the source should be placed at a distant location (far field) to imitate plane waves from the universe, making it difficult to perform the measurement in a laboratory or in a space chamber. Near-field measurement method computes the telescope response to the plane waves from the amplitude and phase measured near the aperture (near field) and enables precise millimeter-wave measurements in a small, controlled environment.