JAXA’s Arase satellite observes the Earth’s radiation belts: analysis of electron fluxes and calibration of Arase’s HEP instrument
Jan. 5, 2024 | Aerospace Project Research Associate, ISAS people
Research Summary
Shortly after launching the first satellites to space, the instruments on-board those spacecraft found intense radiation around our planet. The radiation is caused by energetic charged particles, such as electrons and protons, which are trapped in the Earth’s magnetic field. These regions were named the Van Allen radiation belts. The area with high particle radiation spans from about 1,000 km to 60,000 km from the Earth’s surface. Other planets in the Solar System that have magnetic fields, like Jupiter, also have radiation belts.
The Earth’s radiation belts are an important region to our technology-based society since many satellites, such as communication and navigation satellites, orbit the Earth there. The high radiation environment wears out satellites faster than in other regions, and particularly high-energy particles can cause single-event upsets, such as internal damage or errors in data transmission. Thus, it is important to know how conditions in the radiation belts change. If we observe that an eruption of plasma from the Sun is arriving at Earth, will it cause intense “space weather”? Do satellite operators need to take some action to mitigate the risks? These are the kind of questions that scientist researching the radiation belts want to find answers to.
Scientific measurements of the radiation belts are provided for example by the JAXA’s Arase satellite. Arase has many instruments for measuring the charged particles and the magnetic and electric fields in space. Particle telescopes count the number of particles the satellite encounters at a wide variety of energies. Field detectors and wave analyzers provide information on the magnetic and electric field direction and magnitude, and the occurrence of plasma waves. It is important to understand the waves, since waves interact with the charged particles in the radiation belts and can accelerate them, move them around or cause them to fall into the Earth’s atmosphere.
The radiation belts are split into two regions: the inner belt which is dominated by protons and the outer belt which is dominated by electrons. The number and energy of protons varies slowly from days to years, whereas the electrons in the outer radiation belt are very dynamic. Large changes can occur in only a few minutes. Researchers have found some mechanisms, such as interaction with a type of waves called chorus waves, which can quickly accelerate electrons to high energies. There is also understanding when and where chorus waves will typically show up and how they affect the electrons on average. Nevertheless, it is still difficult to predict in advance what the result will be in a specific event, and the topic is tackled by many scientists. The research approaches vary from analyzing observations and testing theories to performing both small and large-scale simulations.
My research is focused on radiation belt electrons, and I use Arase data to study them. Arase observes electrons with four particle instruments: LEP-e, MEP-e, HEP and XEP. These acronyms stand for the low-energy, medium-energy, high-energy and extremely high-energy electrons experiments. The HEP instrument has both low and high energy detectors named HEP-L and HEP-H. Together, these four instrument suites measure electrons with energies from 10 eV (electronvolts) to 3,000,000 eV. An electron that has energy of 3 MeV (megaelectronvolts) is moving at a speed that is 99% of the speed of light. Such extremely high-energy electrons are commonly observed in the outer radiation belt.
The working principle of particle detectors is that they count each particle that enters the detector. The energy of the particle is also recorded within the bounds of the instrument’s energy resolution. Thus, the preliminary measurement is the count rate as a function of energy. The next step is to process these data into a quantity called particle flux. This is because the geometry and efficiency of each detector is different, such that two different detectors would count a different number of particles in identical conditions. Particle flux describes the number of detected particles per time, energy, area, and solid angle (related to the field of view of the detector). Flux values from different detectors can be compared with each other.
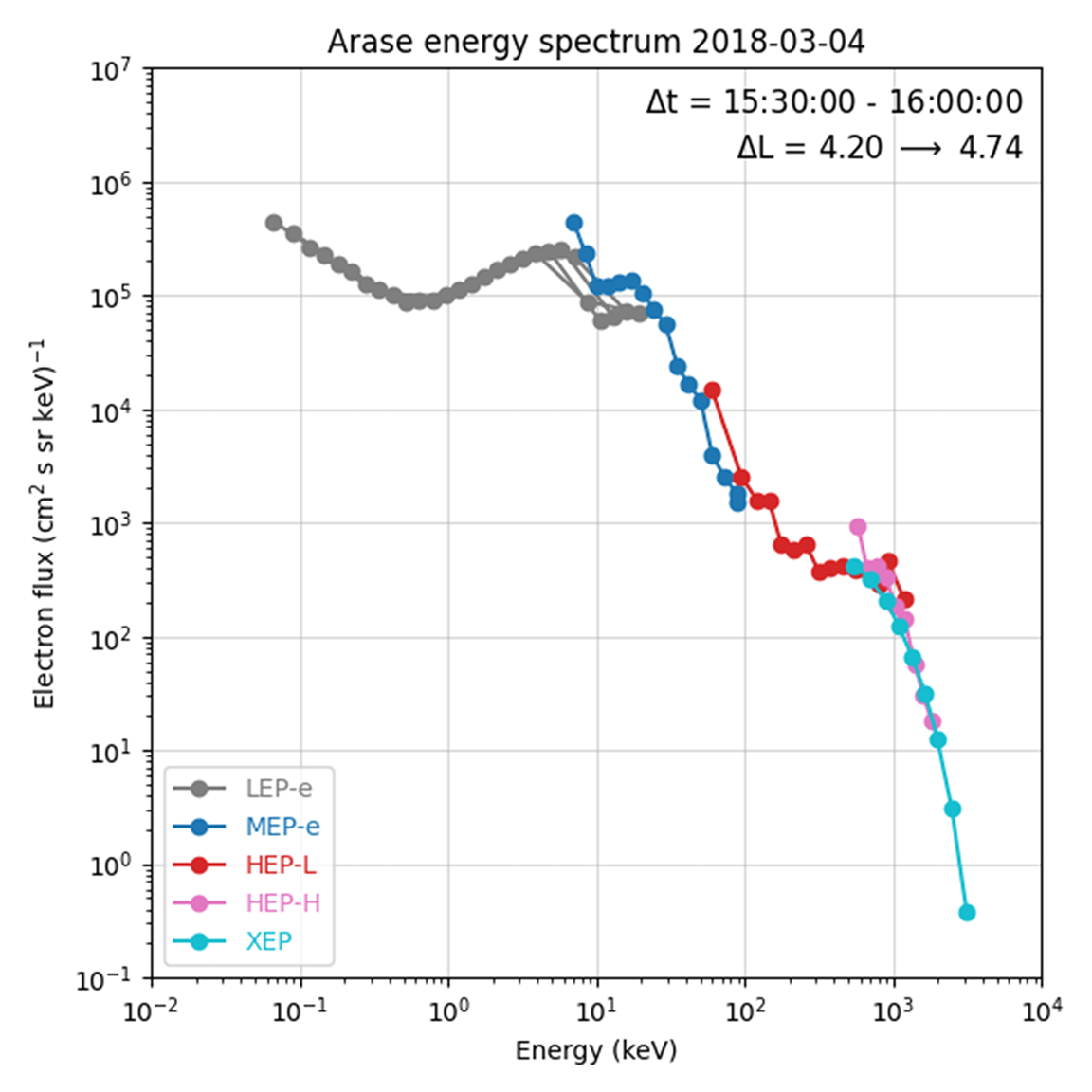
Figure 1 presents Arase electron fluxes as a function of energy during a 30-minute interval on 4th March 2018. Measurements from the LEP-e, MEP-e, HEP and XEP instruments are shown in different colors. The measurements by different instruments agree well with each other. This type of plot is called the energy spectrum. It shows us how flux is distributed in energy. By comparing this figure to the energy spectrum at earlier or later times, we can infer the time evolution of the radiation belt conditions. Arase fluxes also compare well with measurements by other satellites, such as the NASA’s Van Allen Probes (see Miyoshi et al., 2020, https://doi.org/10.1007/s11214-022-00885-4). In Figure 1, the location of the Arase spacecraft is also indicated. In radiation belt physics, researchers typically use “L-shell” for location. L-shell describes the equatorial distance of Earth’s magnetic field lines in units of the Earth’s radius. L-shell of about 4 is in the heart of the outer radiation belt which is a region of interest.
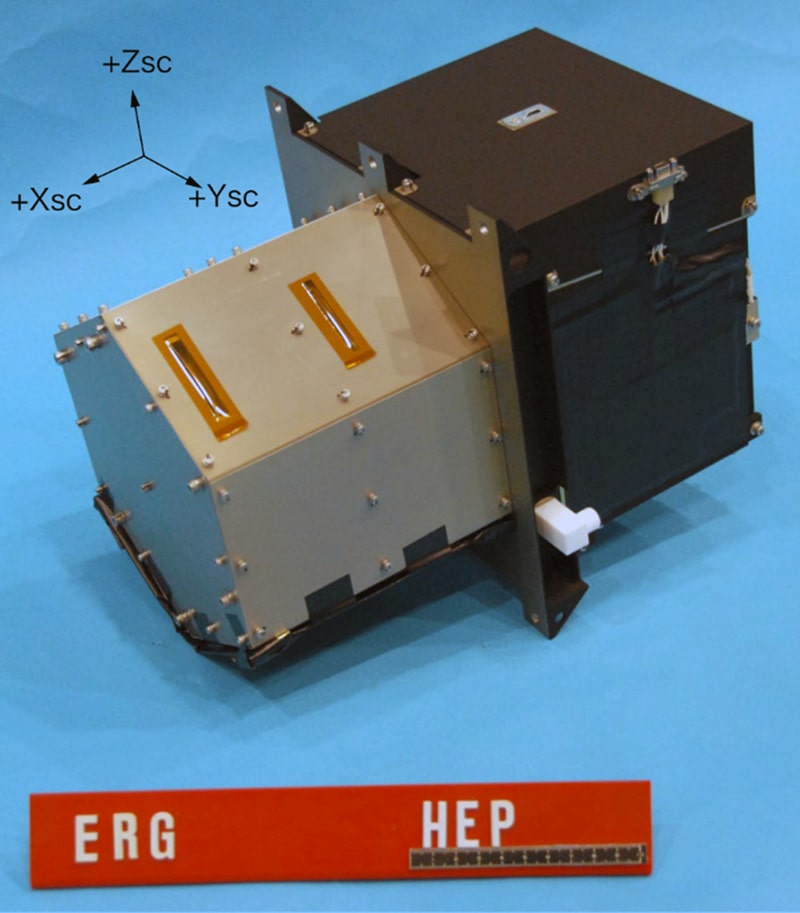
My work in JAXA includes analyzing and calibrating Arase measurements made by the HEP-L instrument. Figure 2 shows a photo of the HEP instrument suite. Arase particle detectors are generally well calibrated, but there are some details still left to analyze. For example, solving what is the contribution of very high-energy electrons in contaminating measurements at lower energies. Instruments are built form material that generally shields them from incoming particles apart from the collimator hole of the instrument. The collimator also has a shield layer before particles reach the detectors in order to select only particles in the correct energy range studied by the instrument. However, very high-energy electrons can penetrate the shielding and the walls of the instrument and deposit part of their energy into the detector which correspond to low-energy electron signatures. Thus, they produce noise in the measurements.
Observations of high-energy electrons by the XEP instrument can be used to estimate the level of this noise. If the flux of energetic electrons is large, the noise is naturally expected to be larger. For a more detailed understanding, simulations of the HEP-L instrument performance have been implemented. A detailed, three-dimensional model of the instrument is placed in the simulation tool Geant4 which tracks the path of a large number of electrons that have been initialized at different energies. The simulation records how much energy each electron deposits to each detector layer and produces a virtual count rate in the instrument’s energy bins. The model result represents an ideal measurement, whereas in the real world noise is always present. Calibration coefficients can be derived by comparing the results with the actual measurements. For example, based on the simulation we can estimate the number of counts observed by HEP-L that are caused by energetic electrons given the amount of flux measured by XEP in each event.
Arase’s HEP particle detector is a state-of-the-art instrument in its capability of distinguishing the incoming direction of electrons by position sensitive detectors. Therefore, the pitch angle of the measured electrons can be accurately determined. The pitch angle is the angle between the magnetic field and the velocity of the electron. The motion of electrons in the radiation belts is strongly constrained by the geomagnetic field: electrons move along magnetic field lines while rotating around them. The geomagnetic field strengthens towards the Earth’s surface, and when the magnetic field magnitude is strong enough, it acts as a mirror to the electrons. Electrons bounce along magnetic field lines between the Earth’s polar regions. The pitch angle determines the location where the electron bounces back and is thus an important quantity in radiation belt research. For example, plasma waves interact differently with electrons based on their pitch angle. Electron fluxes can be binned based on the pitch angle, thus deriving the pitch angle distribution.
Another topic in my work is comparing Arase electron pitch angle distributions with those measured by Van Allen Probes. Since the conditions in the radiation belts vary in location, it is important to first find time periods where these spacecraft are sufficiently close to each other. Such events are called conjunctions. During close conjunctions, satellites should measure the same electron population so that any differences in the observations are assumed to be due to instrumental differences and noise. Such comparisons are beneficial in cross-calibration efforts between science missions.
Currently, Arase is the main scientific satellite with efficient and high-resolution instrumentation monitoring the radiation belts due to the retirement of previous missions. It provides valuable measurements of the radiation belt conditions for both particle fluxes and plasma waves. Continued work on high-tuning the calibration of its instruments will make its measurements even more precise.