It’s hard to catch MeV gamma-ray signals from space! But the Electron-Tracking Compton Camera could the savior! ~ Revealing background events in a balloon experiment and the usefulness of the Electron-Tracking Compton Camera ~
Feb. 26, 2024 | GATEWAY to Academic Articles
The objective of the SMILE group is to observe cosmic MeV gamma rays using our unique gamma-ray imaging detector, the “Electron-Tracking Compton Camera (ETCC)”, and to reveal a picture of the Universe that nobody has seen before. In observing cosmic MeV gamma rays, it is necessary to detect tiny MeV gamma-ray signals within huge amount of background signals. The understanding of these background events is therefore a crucial challenge. This paper reveals that background events are composed of atmospheric gamma rays generated by cosmic rays reacting with atmospheric matter, secondary particles produced by interaction between detector materials and cosmic rays, and radioactive materials within the scintillator detector. Furthermore, we have demonstrated that utilizing the track information of Compton-scattered electrons can effectively remove these background events. This is a significant breakthrough in telescope design and MeV gamma-ray astronomy.
Research Summary
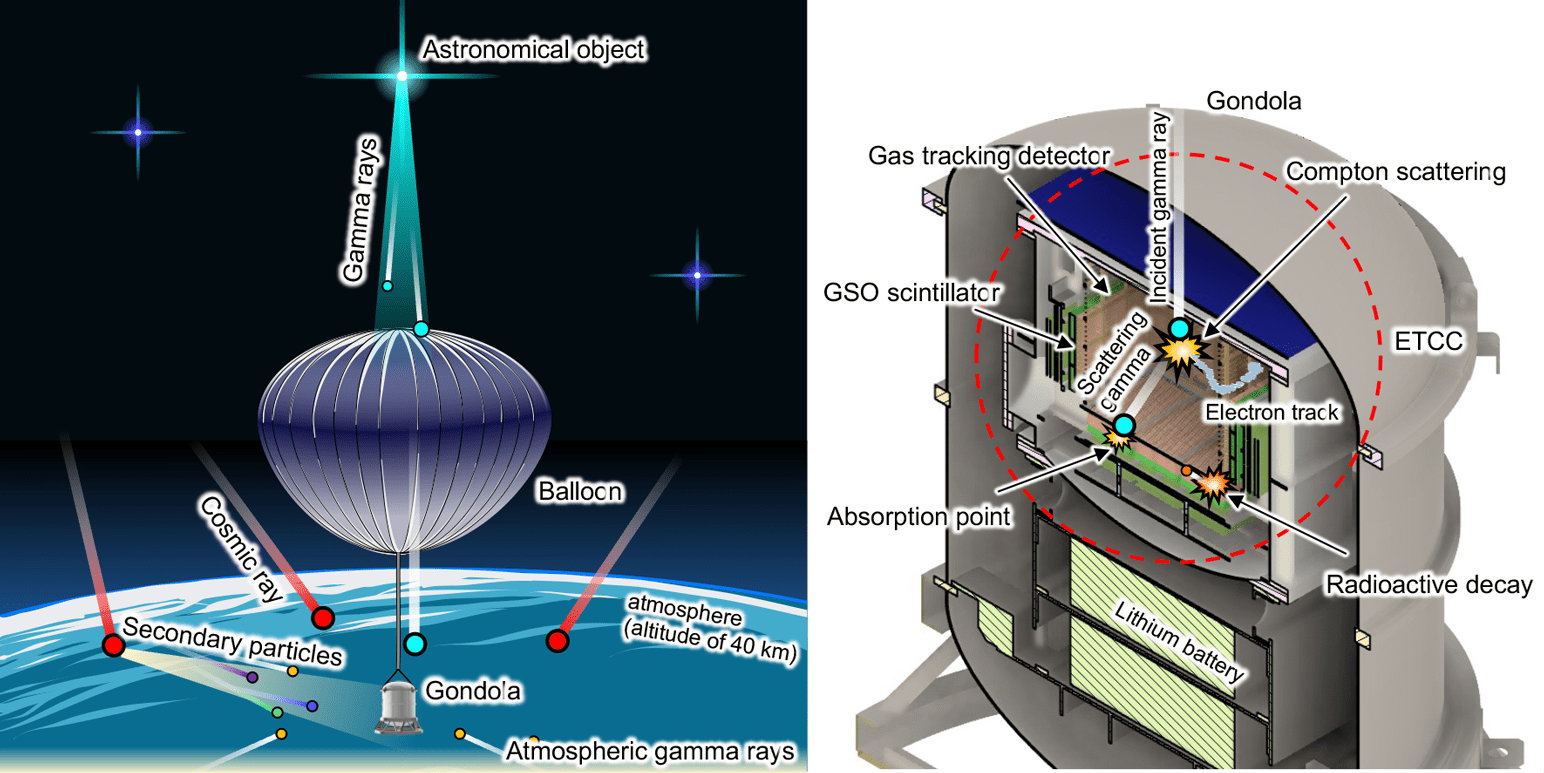
Throughout our history, we have successfully captured cosmic images using a variety of wavelengths, including microwaves, visible light, and X-rays*1. However, there has been little progress in the past 20 years in all-sky imaging using MeV*2 gamma rays, a type of light with a thousand times more energy than X-rays. The main issue is the difficulty in focusing MeV gamma rays, preventing the creation of mirror-based telescopes. Additionally, in balloon or satellite experiments, we encounter a significant amount of noise or background in our images, such as atmospheric gamma-rays from cosmic ray*3 interaction, secondary particles, and gamma rays produced within the detector material (see Fig. 1, left). The COMPTEL*4 experiment, a significant success in observing the Universe using MeV gamma rays, was equipped with the ability to eliminate background events. However, there were more background events than expected, making it difficult to achieve the anticipated performance. The next generation of observations requires gamma-ray imaging detectors that can more efficiently discriminate signals from background events.
To address this challenge, we have developed the Electron-Tracking Compton Camera (ETCC*5) (refer to Fig. 1, right). The key feature of this detector is its ability to measure the recoil direction of electrons that have undergone Compton scattering*6. This allows us to use a method called “alpha angle test” *7 to selectively extract only the gamma rays interacted by Compton scattering. Since most background events originating from cosmic rays or radioactive materials do not have Compton scattering process within the detector, we can efficiently remove these background events.
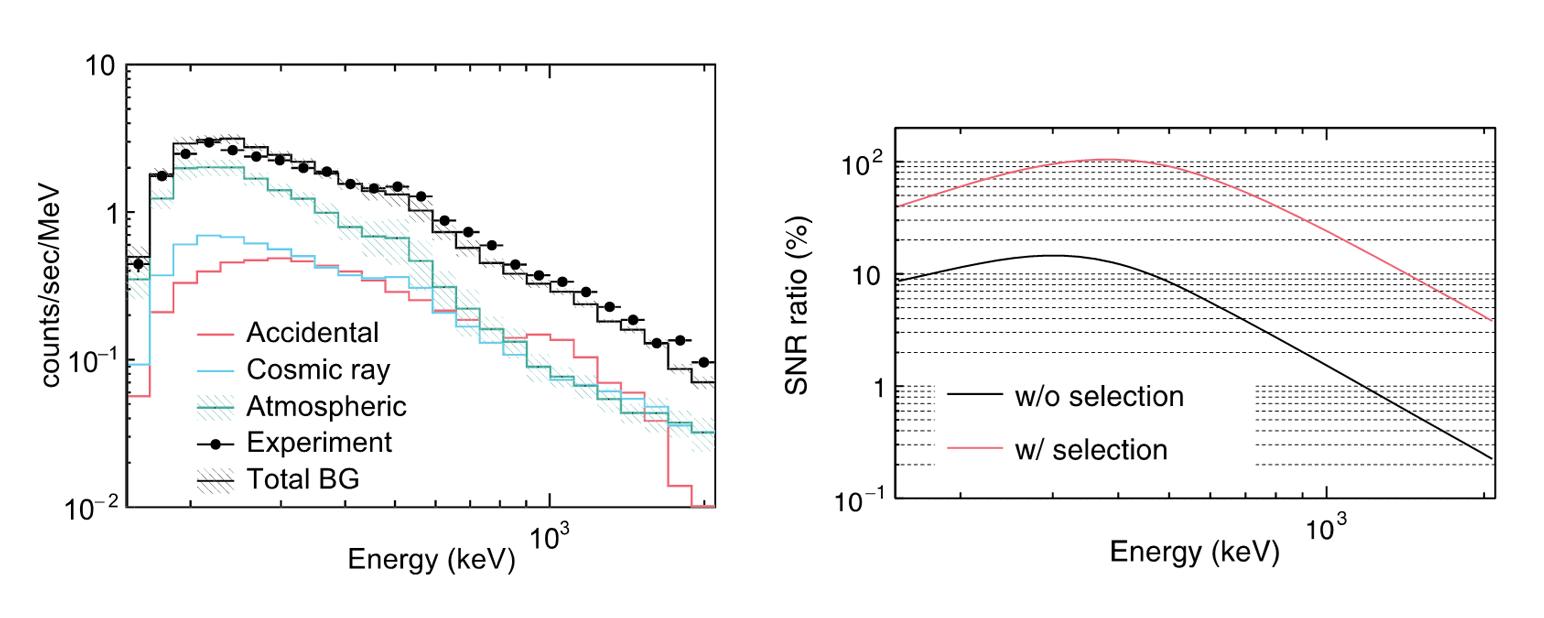
In this study, we analyzed the flight data from the SMILE-2+ balloon experiment conducted in Australia in 2018 using the ETCC, and investigated the background events in the MeV gamma-ray band at an altitude of about 38 km. It is possible to estimate what constitutes the background events through simulation. We carried out detailed modeling of the balloon’s gondola and detector, and estimated the number of events that would be detected by the ETCC using the particle transport simulation Geant4*8. The results of the energy spectrum are shown in Fig. 2, left. The background events in the flight data can be explained by atmospheric gamma rays (green line), gamma rays originating from cosmic rays (blue line), and accidental events (red line). In particular, these accidental events refer to the simultaneous detection of alpha rays from radioactive materials in the GSO scintillator detector*9, that makes up the ETCC, and atmospheric gamma rays. Furthermore, by using simulation data that replicated this experiment, we examined whether the alpha angle test could improve the signal-to-noise ratio and found that, in principle, an improvement in sensitivity of about an order of magnitude is possible (as shown in Fig. 2, right).
From the results of this study, it can be said that the ETCC has the potential to remove many background events in a balloon experiment environment. The cosmic MeV gamma-ray band is heavy with of noise, but the ETCC can clean up the image. It’s going to show us new sides of the Universe that we’ve never seen before.
Terminologies
- *1 Microwaves are electromagnetic waves with wavelengths ranging approximately from 0.001 to 1 meter, visible light has wavelengths of a few hundred nanometers, and X-rays have wavelengths of about 0.01 to 1 nanometer.
- *2 A unit of energy equivalent to one million electron volts.
- *3 High-energy particles that exist in space. About 90% are protons and approximately 8% are alpha particles.
- *4 An experiment aimed at imaging cosmic MeV gamma rays. It was launched in 1991 by NASA and mounted on the Compton Gamma Ray Observatory (CGRO).
- *5 A detector capable of determining the arrival direction of gamma rays. It consists of a gas track detector and a scintillator detector. It records all information when an incoming gamma ray undergoes Compton scattering in the gas detector.
- *6 Compton scattering is an inelastic reaction between electromagnetic waves and electrons. When electromagnetic waves collide with electrons, the waves lose a part of energy while the electrons gain kinetic energy.
- *7 The angle, formed between the scattered gamma ray and the recoil direction of the electron in a Compton scattering, is defined as the alpha angle. The alpha angle can be calculated by solving the equations of motion for Compton scattering using the energy information of the scattered gamma ray and the Compton-scattered electron obtained from the detector. Alternatively, it can be geometrically calculated if the recoil direction of the Compton-scattered electron and the scattering direction of the gamma ray are detected. If the alpha angles obtained by these two methods match, it confirms the occurrence of a Compton scattering event.
- *8 A software for simulating the tracks of particles in matter using Monte Carlo method.
- *9 A radiation detector that uses scintillator materials which absorb radiation and emit fluorescence. GSO is represented by the chemical formula Gd2Si2O7.
Information
Journal Title | PHYSICAL REVIEW D |
---|---|
Full title of the paper | Background contributions in the electron-tracking Compton camera aboard SMILE-2+ |
DOI | https://doi.org/10.1103/PhysRevD.108.123013 |
Publish date | 8 December 2023 |
Author(s) | Tomonori Ikeda, Atsushi Takada, Taito Takemura, Kei Yoshikawa, Yuta Nakamura, Ken Onozaka, Mitsuru Abe, Toru Tanimori, and Yoshitaka Mizumura |
ISAS or JAXA member(s) among author(s) | MIZUMURA Yoshitaka (Department of Interdisciplinary Space Science, Institute of Space and Astronautical Science) |